
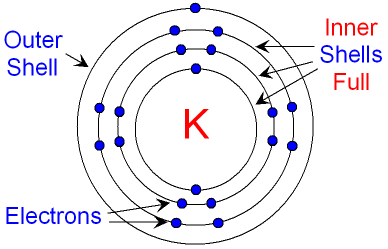
Since energy must be conserved, the energy difference between the two states equals the energy carried off by the photon. The frequency of light emitted is a function of the energy of the transition. In physics, emission is the process by which a higher energy quantum mechanical state of a particle becomes converted to a lower one through the emission of a photon, resulting in the production of light. Similarly, the emission spectra of molecules can be used in chemical analysis of substances. Therefore, spectroscopy can be used to identify elements in matter of unknown composition. Each element's emission spectrum is unique. This collection of different transitions, leading to different radiated wavelengths, make up an emission spectrum. There are many possible electron transitions for each atom, and each transition has a specific energy difference. The photon energy of the emitted photons is equal to the energy difference between the two states. The emission spectrum of a chemical element or chemical compound is the spectrum of frequencies of electromagnetic radiation emitted due to electrons making a transition from a high energy state to a lower energy state. A demonstration of the 589 nm D 2 (left) and 590 nm D 1 (right) emission sodium D lines using a wick with salt water in a flame


The orientational flexibility of SiH₃– can be attributed to the simultaneous presence of a lone pair and (weakly) hydridic hydrogen ligands, leading to an ambidentate coordination behavior toward metal cations.Frequencies of light emitted by atoms or chemical compounds Emission spectrum of a ceramic metal halide lamp. The occurrence of rotator phases α-ASiH₃ near room temperature and the presence of dynamical disorder even in the low-temperature β-phases imply that SiH₃– ions are only weakly coordinated in an environment of A⁺ cations. The reorientational mobility of H atoms in undercooled α-phase is reduced, with relaxation times on the order of picoseconds. ²H NMR revealed that the α-form can coexist, presumably as 2–4 nm (sub-Bragg) sized domains, with the β-phase below the phase transition temperatures established from Cₚ measurements. The first-order reconstructive phase transition is characterized by a large hysteresis (20–40 K). In α-ASiH₃, the SiH₃– anions undergo rotational diffusion with average relaxation times of 0.2–0.3 ps between successive H jumps. Pronounced anharmonicity above 200 K, mirroring the breakdown of constraints on SiH₃– rotation, is also seen in the evolution of atomic displacement parameters and the broadening and eventual disappearance of libration modes in the Raman spectra. The Cₚ(T) behavior is characteristic of a rotator phase transition by increasing anomalously above 120 K and displaying a discontinuous drop at the transition temperature. The transition is accompanied by a large molar volume increase of about 14%. Upon heating the transition occurs at 279(3) and 300(3) K for RbSiH₃ and KSiH₃, respectively. At temperatures below 200 K ASiH₃ exist as hydrogen-ordered (β) forms. The crystal structure of α-ASiH₃ corresponds to a NaCl-type arrangement of alkali metal ions and randomly oriented, pyramidal, SiH₃– moieties. The β–α (order–disorder) transition in the silanides ASiH₃ (A = K, Rb) was investigated by multiple techniques, including neutron powder diffraction (NPD, on the corresponding deuterides), Raman spectroscopy, heat capacity (Cₚ), solid-state ²H NMR spectroscopy, and quasi-elastic neutron scattering (QENS).
